Research & Independent Study
Undergraduate students can conduct intensive, hands-on research for academic credit by taking independent study courses. Independent study can qualify you for Graduation with Departmental Distinction. With some planning, these courses can be a part of several of our signature research programs described below.
Signature Programs
Pratt Research Fellows
Three semesters of independent study, plus summer research.
Katsouleas NAE Grand Challenges Scholars
Flexible curriculum focused on a societal grand challenge. Includes research, courses outside of engineering, training/experience in entrepreneurship and both a global and service learning component.
Smart Home Fellows
One or more semester of independent study focused on smart technology and/or sustainable living.
Find a Research Experience
Browse the gamut, from volunteering in a lab to funded fellowships
Independent Study with Faculty
The opportunities for independent study at Duke particularly rich. To take advantage, you’ll need to do some preparation.
Discuss it with the Director of Undergraduate Studies well before registration opens for the semester. Work to identify faculty you’d like to study with. You can work with faculty from any engineering department.
Start by exploring the research interests of our faculty.

Independent Study Courses
Student | Course(s) |
---|---|
Second Years | EGR Independent Study Courses (Duke NetID required.) |
Third Years | ME 391 & 392 Undergraduate Projects in Mechanical Engineering |
Fourth Years | ME 491 & 492 Special Projects in Mechanical Engineering |
Undergraduate Fellows | ME 394, 493 & 494 Engineering Undergraduate Fellows Projects |
Undergraduate Contacts
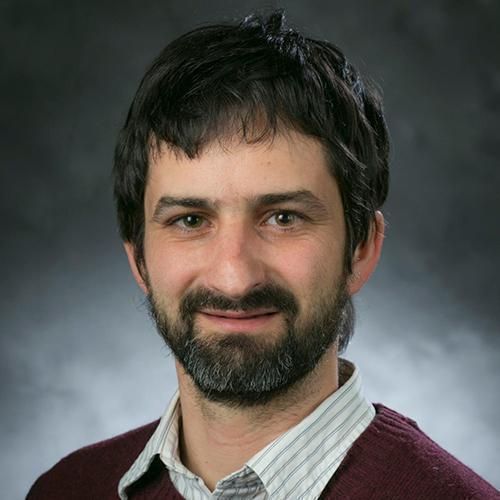
Nico Hotz
MEMS Director of Undergraduate Studies, Associate Professor of the Practice

Sophia Santillan
Associate Director of Undergraduate Studies, Associate Professor of the Practice in the Thomas Lord Department of MEMS